Recent publications related to this project:
Cummings, B., Li, Y., DeCarlo, P. F., Shiraiwa, M. and Waring, M.*: Indoor aerosol water content and phase state in U.S. residences: Impacts of relative humidity, aerosol mass and composition, and mechanical system operation, Environ. Sci. Processes Impacts, 10.1039/D0EM00122H, 2020.
Yeoman, A., M. Shaw, N. Carslaw, T. Murrells, N. Passant, and A.C. Lewis, Simplified speciation and atmospheric VOC emission rates from non-aerosol personal care products, 30, 459-472, Indoor Air, 2020.
Kruza, M., McFiggans G., Waring M.S., Wells J.R., Carslaw N. Indoor secondary organic aerosols: towards an improved representation of their formation and composition in models. Atmos. Environ., 240, 117784, 2020a.
Wang, Z, S. F. Kowal, N. Carslaw, T. F. Kahan, Photolysis driven indoor air chemistry following cleaning of hospital wards, Indoor Air, 2020, https://doi.org/10.1111/ina.12702.
Won, Y., Lakey, P. S. J., Morrison, G., Shiraiwa, M. and Rim, D.*: Spatial Distributions of Ozonolysis Products From Human Surfaces In Ventilated Rooms, Indoor Air, 10.1111/ina.12700, 2020.
Wang, W., Ezell, M. J., Lakey, P. S. J., Aregahegn, K. Z., Shiraiwa, M.* and Finlayson-Pitts, B. J.*: Unexpected formation of oxygen-free products and nitrous acid from the ozonolysis of the neonicotinoid nitenpyram, Proc. Natl. Acad. Sci. U.S.A., 117, 11321-11327, 2020.
Von Domaros, M., Lakey, P. S. J., Shiraiwa, M.* and Tobias, D. J.*: Multiscale Modeling of Human Skin Oil-Induced Indoor Air Chemistry: Combining Kinetic Models and Molecular Dynamics, J. Phys. Chem. B, 124, 18, 3836-3843, 2020.
Mattila, J. M., Lakey, P. S. J., Shiraiwa, M., Wang, C., Abbatt, J. P. D., Arata, C., Goldstein, A. H., Ampollini, L., Katz, E. F., DeCarlo, P., Zhou, S., Kahan, T. F., Cardoso Saldaña, F. J., Hildebrandt Ruiz, L., Abeleira, A., Boedicker, E. K., Vance, M. E. and Farmer, D. K.: Multiphase chemistry controls inorganic chlorinated and nitrogenated compounds in indoor air during bleach cleaning, Environ. Sci. Technol., 54, 1730-1739, 2020.
Youngbo Won, Michael Waring, Donghyun Rim: Understanding the spatial heterogeneity of OH due to indoor photolysis of HONO using Computational Fluid Dynamics (CFD) simulations, Environ. Sci. & Technol., 53, 24, 14470-14478, 2019.
Morrison, G.*, Lakey, P. S. J., Abbatt, J., Shiraiwa, M.*, Indoor boundary layer chemistry modeling, Indoor Air, 29 (6), 956-967, 2019.
Zhou, S., Hwang, B. C. H., Lakey, P. S. J., Zuend, A., Abbatt, J. P. D.* and Shiraiwa, M.*: Multiphase reactivity of polycyclic aromatic hydrocarbons is driven by phase separation and diffusion limitations, Proc. Natl. Acad. Sci. U.S.A., 116(24):11658-11663, 2019.
Lakey, P. S. J., Morrison, G. C., Won, Y., Parry, K. M., von Domaros, M., Tobias, D. J.*, Rim, D.*, and Shiraiwa, M.*: The impact of clothing on ozone and squalene ozonolysis products in indoor environments, Commun. Chem., 2, 56, 2019. (associated blog post in Nature Research Community), 2019.
Shiraiwa*, N. Carslaw, D. J. Tobias, M. Waring, D. Rim, G. Morrison, P. Lakey, M. Kruza, M. von Domaros, B. Cummings and Y. Won, Modelling Consortium for Chemistry of Indoor Environments (MOCCIE): Integrating chemical processes from molecular to room scales, Environ. Sci. Processes Impacts, 21, 1240 – 1254, 2019. (Selected as one of the Best Papers 2019)
Cummings, B. E. and M. S. Waring*: Predicting the importance of oxidative aging on indoor organic aerosol concentrations using the two-dimensional volatility basis set (2D-VBS), Indoor Air, 29(4): 616-629, 2019.
Fang, Y., Lakey, P., Riahi, S., McDonald, A., Shrestha, M., Tobias, D. J.*, Shiraiwa, M.* and Grassian, V.*: A Molecular Picture of Surface Interactions of Organic Compounds on Prevalent Indoor Surfaces: Limonene Adsorption on SiO2, Chem. Sci., 10, 2906-2914, 2019. (highlighted as ChemSci Pick of the Week), 2019.
Fang, S. Riahi, A. T. McDonald, M. Shrestha, D. J. Tobias*, and V. H. Grassian*, What Is the Driving Force behind the Adsorption of Hydrophobic Molecules on Hydrophilic Surfaces?, J. Phys. Chem. Lett., 10, 468-473, 2019.
Rim, D.*, Gall, E. T., Ananth, S., & Won, Y.: Ozone reaction with human surfaces: Influences of surface reaction probability and indoor air flow condition, Build. Environ., 130, 40-48, 2018.
Overview
This project is a continuation of the MOCCIE modelling consortium that brings together modelling experts from different fields to develop comprehensive, integrated physical-chemical models that include a realistic representation of gas-phase and surface chemistry in indoor environments and how occupants, indoor activities and building conditions influence indoor processes. The MOCCIE models represent the chemistry of indoor environments over a wide range of different spatial and temporal scales from sub-nanometer to tens of meters and from sub- nanosecond to days. For MOCCIE2, we have two new groups in the consortium to expand our expertise further (Fig. 1).
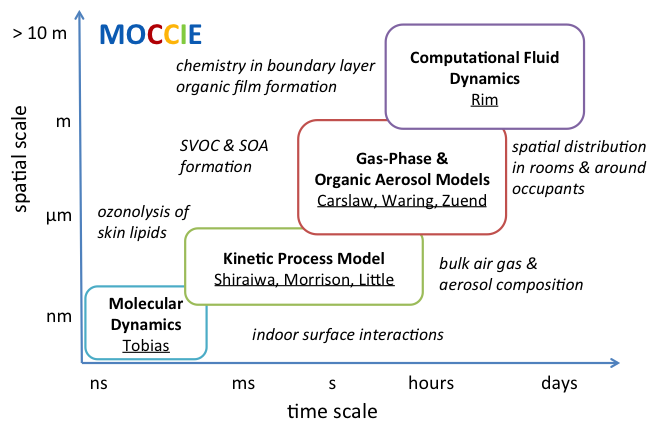
MOCCIE was initiated in 2017 to bring together modeling experts to: 1) assess gaps in our fundamental understanding of indoor chemistry processes; 2) help guide measurements through identification of parameters responsible for model uncertainties, indicating key species with predicted concentrations, and improving design of experimental/field studies; 3) assist in interpretation of data from field studies and experiments including the House Observations of Microbial and Environmental Chemistry (HOMEChem) study. Eventually such models can be used to predict when indoor air chemical processes may adversely affect health and well-being, to aid in the design and effective operation of buildings, and to inform the evaluation and redesign of consumer products used in homes.
We continue to address the following research questions:
- Can we understand indoor chemistry well enough to predict it quantitatively with computer models of the fundamental chemical and physical processes?
- What are the major uncertainties that currently exist in these models?
- What experiments/field measurements do we need to improve/evaluate our models?
Research
Modeling studies are conducted by MOCCIE to explore three relevant and highly important themes for indoor air quality: 1) Impact of reactions of indoor oxidants with skin and clothing on indoor chemistry; 2) Impact of VOC chemistry, organic aerosol behavior, and SOA formation on indoor air quality; 3) Mass transport and partitioning of species to indoor surfaces and materials. Interactions of indoor oxidants with skin, cleaning-related emissions of VOCs, and surface interactions are important processes, each strongly affecting chemical composition and distribution in indoor environments. These themes are useful as they encompass the necessary range of spatial and temporal scales that allow us to employ and test the models for relevant indoor scenarios. In the development of MOCCIE, we initiated integration of a variety of models with the aim of working towards the development of an overarching comprehensive indoor chemistry model, which remains our goal through MOCCIE2. The work is organized into the following work-packages:
WP1: Impact of reactions of indoor oxidants with skin and clothing on indoor chemistry
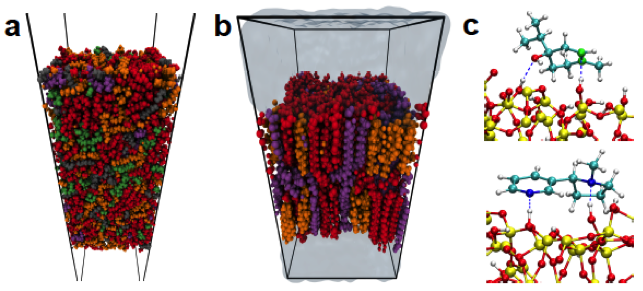
MD simulations will be applied to provide new mechanistic insights and predict thermodynamic and kinetic parameters for the transport of ozone and volatile squalene oxidation products through the outermost layers of skin. The Tobias group has constructed and equilibrated atomistic, multi-component models of skin oil (Fig. 2a) and the lipid matrix of the stratum corneum (SC) (Fig. 2b). Using MD simulation protocols developed in their previous study of ozone uptake and transport in squalene, they will calculate diffusion constants, surface mass accommodation coefficients, Henry’s law constants, and desorption lifetimes for ozone and ozonolysis products in skin oil, as well as transport kinetic parameters for the same chemical species in SC lipids, which will be used to update KM-SUB-Skin and KM-SUB-Skin-Clothing. We will continue to develop these models by treating the ozonolysis of squalene and other skin components with a more complex mechanism by explicitly treating chemistry of Criegee intermediates. We continue to work on expanding existing models of uptake and chemical transformations on porous materials such as clothing and other textiles, paint, wood and paper. We will include reactions of ozone and other oxidants with VOCs, drawing on measurements made by the Morrison group for surface reactions of ozone with terpenes. Further, the Morrison group will develop models of “deep reservoirs” that are present in materials indoors.
By connecting KM-SUB-Skin-Clothing with the INDCM model that can treat emission from skin and breath, we will simulate field measurements of key squalene ozonolysis products such as 4-OPA, 6-MHO, and acetone. The Waring group will further develop their 2D-VBS model to include OA emissions by ozone reacting with humans, using these results to estimate an emission rate of OA from human-ozone deposition, informed by KM-SUB-Skin-Clothing. OA owing to oxidation of breath emissions will also be included based on INDCM simulations.
The CFD model will be further applied to predict the spatial distributions of primary and secondary reaction products around occupants under varied building operating conditions (Fig. 3) and to examine how skin and breath emissions drive pollutant dynamics around a human body. The results will reveal how human breath containing acetone, isoprene, and ethanol influence ozone chemistry near the human surface and thereby breathing zone concentrations. Comparison of the results with a well-mixed box model will highlight the near-human pollutant dynamics and roles of human occupants in indoor chemistry.
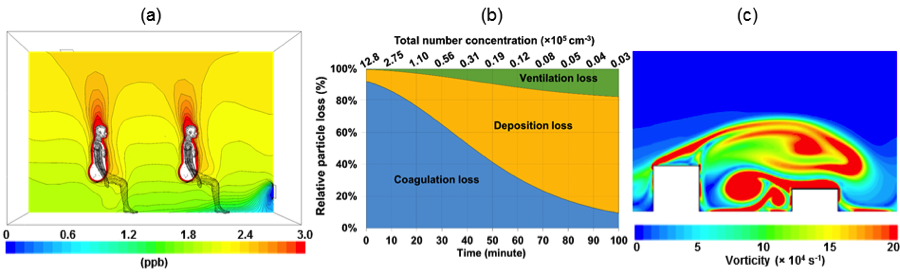
WP2: Chemical evolution of indoor organics
We will continue our work on the gas-to-particle partitioning processes for the terpenes. We now have a mechanism that provides the 2D-VBS model with input data for SOA-aging and we will continue to work on this aspect, further developing it to understand HOMEChem data. Using the results from the Monte Carlo analysis currently being carried out, we will aim to work with experimentalists that can help us to reduce our main model uncertainties.
Following the McDonald et al. (2018) study that highlighted the importance of indoor VOCs for outdoor inventories, we will use the INDCM to explore how indoor composition can affect what ends up outdoors: some VOCs will likely stay in the same form outdoors as emitted indoors if they are not very reactive, but some may have been through several oxidation steps. We will develop statistical models for predicting distributions of air exchange that result from occupant behavior with a dataset the Morrison group has generated on window opening behavior. These models will be used as input to 2D-VBS and INDCM, helping predict input such as particle and gas penetration.
New developments will be incorporated into the 2D-VBS OA model, focusing strongly on outdoor-to-indoor transport and transformation of aerosol air pollution. Outcomes will be predicted using a developed dataset of outdoor OA and inorganic components from EPA Chemical Speciation Network sites in 16 US cities to explore regional and seasonal variation. Also, our 2D-VBS assumes instantaneous equilibrium for OA aerosol and gas phase systems, and we will incorporate kinetic considerations to relax this assumption to account for the effect of particle phase state. The kinetic model coupled with AIOMFAC will evaluate effects of phase state and non-ideal mixing on SOA partitioning.
The 2D-VBS predicts OA evolution with transformation kernels for OH-induced functionalization and fragmentation, and we will compare the OA evolution from our kernels with results from the INDCM for OH-induced aging of fresh SOA from α-pinene and d-limonene ozonolysis to better constrain kernels. In chamber experiments, SOA formation yields are influenced by loss of vapors to surfaces. Indoor surface-to-volume ratios are high (e.g. 3 m-1), so we will include this route of vapor loss to indoor surfaces in the 2D-VBS model. This vapor loss route will have potential implications on reducing the amount of realized SOA formation indoors, and increasing growth of indoor surface films (see WP3). We will also estimate VBS-based emission rates of cooking aerosol, measured during HOMEChem.
The Rim group will establish an indoor particle dynamic model that can analyze detailed indoor aerosol transformation processes (i.e., coagulation, deposition, ventilation) considering building operating conditions (Fig. 3(b)). The model will provide a basis to examine time- and size-dependent particle loss mechanisms of the measurement data (e.g., HOMEChem). In addition, we will improve OH treatment in CFD simulations. Currently HONO is the only source of OH, so if part of a room is in the dark, there is no OH formed. We will use the INDCM to produce a reduced mechanism to use in the CFD model that will account for the impact of ozone-terpene reactions on OH formation.
WP3: Mass transport and partitioning of species to indoor surfaces
A combination of force field-based and ab initio MD simulations will be applied to investigate the interactions of VOCs commonly found indoors with model indoor surfaces, including hydroxylated silica (glass), gypsum (drywall), and TiO2 (paint). We will consider: dihydromyrcenol and linalool, found in personal care products; terpineols (various constitutional isomers), found in cleaning products, perfumes, and cosmetics; p-cymene, found in kitchen emissions and essential oils; and nicotine and propylene glycol, from tobacco products. These compounds possess a variety of functional groups that are expected to produce a variety of modes of interaction with indoor surfaces (Fig. 2c).
We will investigate the chemistry occurring in the boundary layer adjacent to indoor surfaces using an analytical approach and the newly developed kinetic multi-layer model for boundary layer (KM-BL). We will continue to enhance our current model of boundary layer chemistry to include a wider range of the most important gas-phase reactions. In addition, we will include aerosol dynamics such as Brownian motion and SVOC partitioning that are anticipated to result in unique deposition and emissions behaviors at indoor surfaces. The formation and growth of organic films will be included in KM-BL, building on work by the Little group. An important model parameter is the surface/air partition coefficient (Ks) which depends on the specific chemical as well as on organic film and surface characteristics, and may vary as the film grows. Ks will be estimated by experimental data acquired by the Little group in a range of chamber experiments that includes the kinetic buildup of SVOCs on stainless steel and glass surfaces, as well as potential variations in surface roughness, temperature, humidity and ozone. We will evaluate the influence of these factors on the formation and growth of surface films comprised largely of low volatility organic compounds. These data sets will extend and validate Little’s surface film model and KM-BL. MD simulations will also be applied to specific experimental systems to provide fundamental kinetic and thermodynamic parameters for film models.
The Rim group will visualize transport of heat, mass, and momentum in indoor surface boundary layers. The CFD simulation can describe how surface roughness and thermal characteristics influence mass transfer and gas partitioning of indoor oxidants and organic compounds in the surface boundary layer (Fig. 3c). The particular chemicals to be simulated are OH, O3, NO2, and SVOCs due to their important roles in indoor surface chemistry. The fundamental thermodynamic and kinetic parameters acquired from indoor boundary layer and surface modeling will be integrated into the CFD model to simulate complex chemistry occurring in the boundary layer depending on the surface characteristics (e.g., carpet vs. vinyl flooring) and indoor airflow conditions.
Principal Investigators
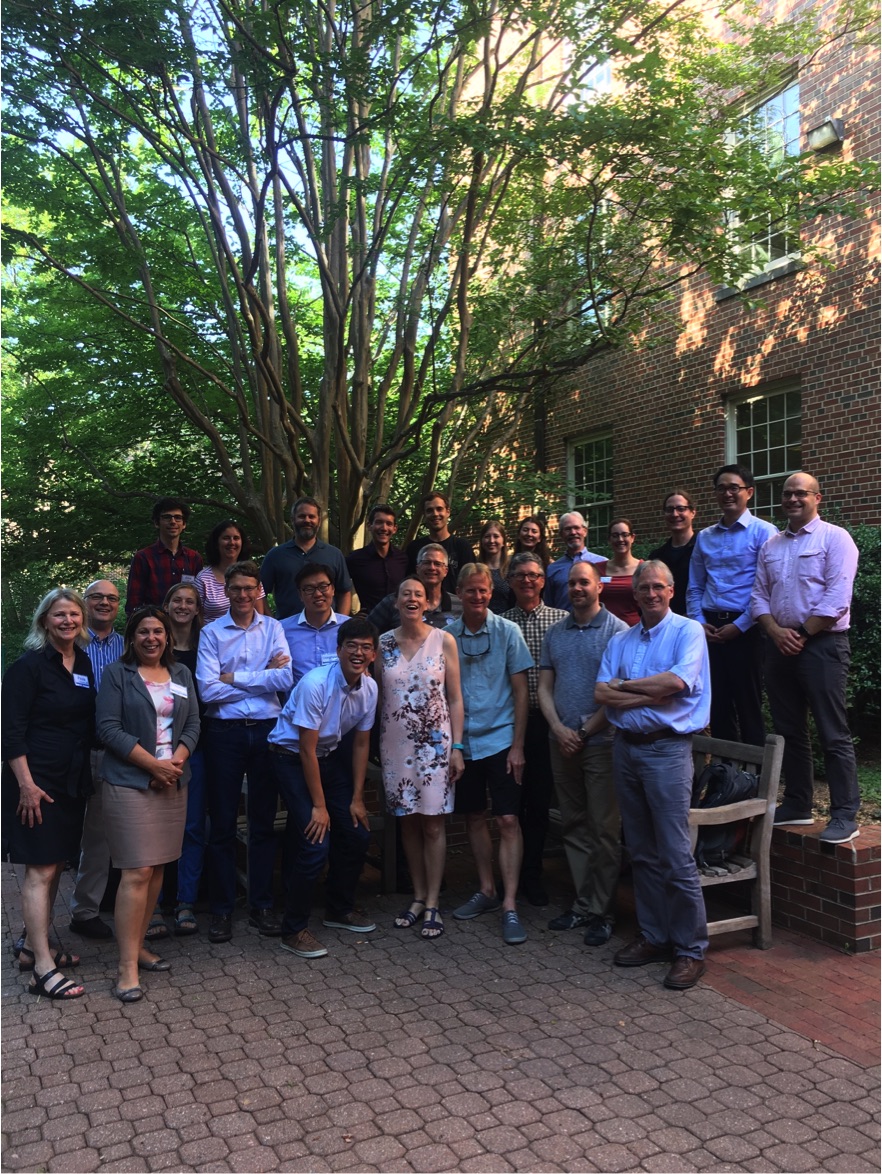
Manabu Shiraiwa is Associate Professor in the Department of Chemistry at UCI, USA
Nicola Carslaw is Reader in Air Pollution in the Department of Environment and Geography at the University of York, UK.
Co-Investigators
John Little is a Professor in the Department of Civil & Environmental Engineering at Virginia Tech, USA
Glenn Morrison is Research Professor at the University of North Carolina.
Donghyun Rim is Assistant Professor in the Architectural Engineering School at Penn State University.
Doug Tobias is Professor of Chemistry at the University of California, Irvine.
Michael Waring is Associate Professor in the Civil, Architectural and Environmental Engineering School at Drexel University
Andi Zuend is Associate Professor in the Department of Atmospheric & Oceanic Sciences, McGill University, Canada
Students/post-doctoral researchers
Bryan Cummings, Civil, Architectural and Environmental Engineering School at Drexel University
Michael von Domaros, Department of Chemistry, UCI
Clara Eichler, Department of Civil & Environmental Engineering at Virginia Tech
Elianna Frank, Department of Chemistry, UCI
Magdalena Kruza, Department of Environment and Geography at the University of York
Pascale Lakey, Department of Chemistry, UCI
Youngbo Won, Architectural Engineering School at Penn State University